#11: Watt's the deal with renewable energy
We need more solar & wind. But are they really that cheap, clean, and green?
[Author’s note: I really think this is an incredibly important topic to understand and I learnt so much in doing the research for this piece. This is also the first piece that uses the base layer concept to analyze a sector/technology, so I highly recommend reading newsletters #2, #6, and #10 before or alongside this.
The goal is not to give a definitive judgement on renewable energy but to provide context to help analyze what we hear about in the news constantly (especially about cost!!)
Both the pro and anti-renewables narrative get so much wrong, and hopefully this piece helps clear some of that and provides a clear framework for thinking about the rest.
I planned to cover electrification in this because it is inseparable from renewable energy but I will just have to do a separate piece on it next.
Please share with your networks & reach out with any thoughts/comments/feedback!]
There is so much to say about this topic. Over the past decade, it has become a part of political correctness almost to be “pro-renewables”. For others, especially recently, it has become an important “I’m a contrarian” marker to be “anti-renewables”. Many on both sides wear it as a badge of honor, armed with the fervent belief that they are doing what is best for human flourishing.
As with the oil primer, I do not want to create a false equivalency between the two positions. We must not only deploy renewable energy (RE from here on), but at an unprecedented scale and breakneck speed.
However, as you may realize is a common theme in this newsletter, to properly and sustainably achieve something, we must first holistically understand it, lest our simplifications and misgivings provide fuel to those looking to keep the status quo going.
If you have ever shared a comment or image saying “solar & wind are so cheap this is a no brainer”, you’re going to want to read this!
TLDR:
Solar & wind aren’t as cheap as the mainstream narrative says.
They are re-buildable, not renewable. They are not green, just green-er than fossil fuels.
There are no easy answers here. The complexity of choices, the scale & speed of development, the level of planning and infrastructure development, etc. required means there is a wide range of possibilities.
In this piece, I provide an overview of the RE sector. It’s quite a broad space with technologies across maturity spectrum, but here I focus on solar and wind, leaving out hydro, nuclear, and some of the emerging technologies.
[Note: Most of the water bodies in the world where hydropower can be developed is already being used so limited opportunity to increase capacity. Nuclear is more complicated — I am pro-nuclear but it warrants a separate piece.
Tip: Every time you hear renewable energy, always check what’s under the hood. 60% of the EU’s renewable energy comes from biomass, which is the burning wood pellets from fast growing trees. Ecologically terrible & not renewable per se. For other countries with a high RE %, like Costa Rica, the majority is made up on existing hydropower.]
There was too much to talk about in this piece from a technology and cost perspective so I couldn’t analyze the geographic and geopolitical dimensions of RE. I also could not discuss electrification and the grid, without which it is impossible to sufficiently understand RE. Both these covered in the next piece!
The 3 sections covered in this piece are:
The basics of RE
Energy quality of RE (cost, reliability, density, EROI)
What’s in a name — how renewable is it?
I. The basics
Renewable energy installed capacity is soaring, with 83% of all newly installed energy capacity being renewable.
Global investment in the energy transition, of which RE is the biggest component, has crossed $1trillion.
Despite this, a sobering reminder that RE is not replacing FFs, it is simply meeting our additional energy needs. There is no energy transition!
Why renewable energy?
Too many times we get into analyses and debates without clearly defining the context, which leads to all sorts of miscommunications. In the energy context, let’s not forget that fossil fuels (FFs) are an almost miraculous form of energy, perched at the top of the energy quality framework. Their unique qualities have allowed us to build this vast, complex, and globalized civilization.
So why should we then care about RE?. There are 3 main reasons:
Decarbonization: It comes as no surprise that this is probably the biggest driver. FFs are causing climate change through CO2 and methane release, they cause air pollution through the release of toxic fumes, their extraction causes ecological damage, and so on.
Energy security: As geopolitical risks increase, the global supply chains that moves FFs from producers to refiners to consumers could become more strained (remember, FFs are a relatively centralized commodity geographically). RE instead provides a more decentralized way to meet energy demand. This is partly why even some of the anti-climate people in the US supported the Inflation Reduction Act.
Declining FF availability: The debate over “peak oil” — which is the date when global oil production peaks — has been raging since the mid-20th century. Horizontal drilling, fracking, shale oil, etc. have all helped boost oil and natural gas supply over the past decade. But I think there is a largely uncontroversial narrative that, barring any miraculous technological change, our oil supply will be (maybe significantly) lower by 2040-2050. Key things to remember here:
Supply of commodities doesn’t depend on how much is stored in the ground but on how much can be accessed at current price and technology levels. Accessing deeper reserves means higher costs, and hence higher prices.
Energy consumption (which for our civilization has meant FF consumption) has a 1:1 relationship with GDP, human development, etc.
If we are indeed running out of cheap FF energy, there is a serious need to find alternative energy sources.
[Unconventional oil is what we extract from harder to access reserves using fracking and other costly methods. More details in the oil primer]
What is renewable energy?
Renewable energy, defined here as solar & wind, is a way for us to convert solar energy from the Sun and the energy in wind currents on Earth to generate electricity. Without getting into the technical details, here’s what you need to know:
Solar energy: When the sun is shining and the solar panel receives sun rays, the energy particles (photons) carried in the rays interact with the material that makes up the panel (mostly silicon) to generate electric current. ~20-30% of the energy that hits the solar panel gets converted into electricity; rest is lost as heat. All solar panels create DC electricity so an inverter is needed to convert that into AC. Then the electricity is either directly consumed (e.g. by a house or a factory), sent into the grid, or stored in a battery.
Panels need to be installed in areas with high exposure to sunlight year round and the angle of installation needs to maximize sun exposure.
Solar panels are quite modular, meaning they can be installed as a single panel or stacked to create power plants. They can also be installed in a variety of locations, ranging from rooftops to out in the fields.
They usually last for 20-25 years.
Wind energy: When the wind blows, the energy carried in the currents rotates the large turbines. This rotation spins the attached generator which then create electricity. ~20-40% of the wind energy gets converted into electricity. No inverter is required since the electricity is already AC.
These turbines are large in wingspan, to maximize the area impacted by the winds, and quite tall, in order to get higher windspeeds. Therefore, they are not as modular, meaning they cannot be installed in smaller sizes (e.g. rooftops). However, they can still be installed in a variety of locations, including in the middle of the oceans (offshore wind turbines) and don’t suffer from the same temporality that solar powers faces since the wind can blow all day.
They also last ~20 years and require more maintenance.
Primary Energy Myth
Before we assess the pros and cons of RE, it is imperative to understand that any analysis that does an apples-to-apples comparison with fossil fuels is inherently insufficient. This is really important and something many renowned energy experts like Vaclav Smil also got wrong until quite recently.
Most RE energy analyses assume that these resources have to replace the total primary energy amount of that fossil fuels produce. Primary energy is total amount of energy consumed at the start of the value chain (before losses, inefficiencies, etc.).
However, whether it is in burning gasoline in cars or coal in power plants, 50-80% of energy in the current system is lost as heat (65% of energy in the US is lost, as shown below).
RE does not have this problem since there is no fuel being burnt — it only has to replace final energy that we use as “useful work”. This does not mean that in a fully renewable world our energy consumption will automatically be 50-80% lower because there are nuances for each sector and the system has to be (re)built in a particular way, but the takeaway is that due to lower heat loss, RE has to replace a smaller energy amount that we currently use (estimates 20-30% smaller).
This requires electrification. For example, replacing gas heaters with heat pumps, diesel trucks with electric trucks, etc. An EV is ~4x more efficient than a combustion engine car because the latter has high heat loss when converting from one energy source to another, while EVs simply use the stored electric energy.
Hence, if you see an analysis that is talking about RE having to replace the full FF primary energy amount, know that it’s automatically misleading.
II. Energy quality analysis of RE
Recap from newsletter #2: There is this myth that 1J of energy from one source can simply be replaced by 1J from another source. Instead, each source has its own features that lead to a certain quality score for that 1J of energy. Here’s the framework below:
1. Reliability (and cost)
This is the big one! If you talk positively about RE a few times, you are bound to be jumped on by someone talking about how these energy sources are not reliable.
“What happens when the sun sets or the wind stops blowing" blah blah. As if this is some new insight that no one has thought off before.
However, I am compelled to say that in the interest of fairness, there are way too many people who tout RE as some techno-utopian dream, again falling for that 1J = 1J myth I talked about above.
The reality is that when it comes to reliability, most experts are obviously aware of the unique challenges that RE face (so it is not some “gotcha” point) but most onlookers, climate-tech folk, and policymakers need to understand the specifics.
This is intimately tied with the cost point, because too many people fall for the “look how cheap solar is, let’s go full steam ahead” argument.
Let me explain.
What is reliability? In short, it is the ability for supply to meet demand 24/7. This is particularly true for electricity, but also applicable to the energy demands for industries and so on. We live in a world where everything is tied to the centralized energy system: heating/cooling, electric devices, transportation, functioning hospitals, etc. Therefore, when reliability standards are not met, the results range from inconvenient (uncharged phones, spoilt food, etc.) to catastrophic (life-saving machinery in hospitals not working).
Fossil fuels are special because they are easy to store. They can sit around until you need them; therefore, excluding external challenges related to distribution and transmission, they are near perfectly reliable. In the electricity sector, they are known as dispatchable resources, reading to be started, stopped, or ramped up/down as needed.
RE sources are of course not that reliable. Solar doesn’t work on a cloudy day or after sunset, and the turbines don’t rotate when the wind stops blowing. You can see below that solar starts around 5-6am, reaches its peak early afternoon, and then declines till it stops at ~9pm (this of course varies by location, time of the year, daily weather, etc.). For wind it is more interesting to look across the month because some days the wind is strong and consistent while others it is not.
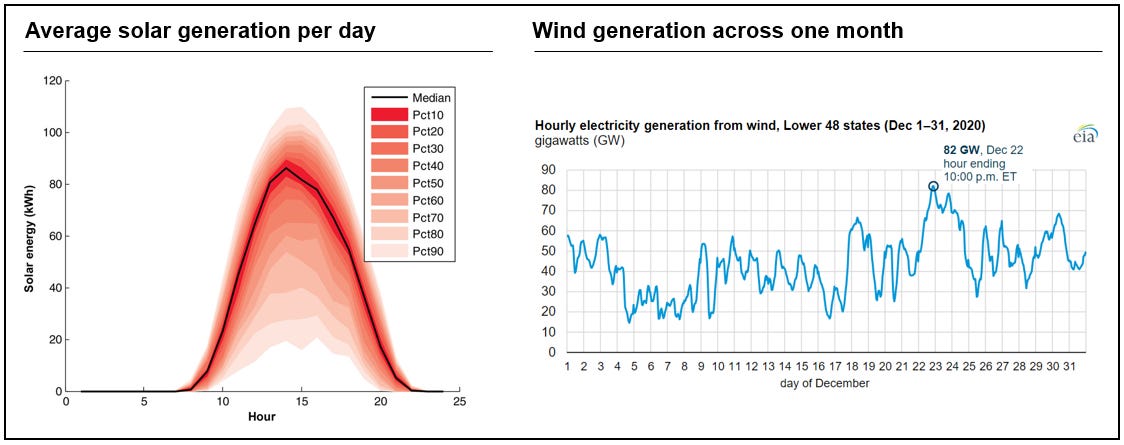
The demand profile looks something like this: it picks up in the morning, then has an afternoon stabilization, and then increases again in the evening more declining into the night. Regardless of where you are in the world, it probably looks like this because we all start consuming more when we wake up, then are usually in offices or schools throughout the day (where there is a lot more collective use of energy) and then come home in the evening to turn on our individual ACs, TVs, etc.
It also makes sense that summers and winters are a lot more energy-intensive because of heightened heating/cooling demand.
Fun fact: The UK electricity utility provider realized that at a particular point in the evening, demand would suddenly ramp up. It realized that was happening because a popular show on cable TV would have its commercial break at that point and everyone would turn on their kettle or microwave or whatever at exactly the same time!
Reliability is simply the ability to match this demand to the supply curve of all energy resources. FFs are controllable and flexible (e.g. simply burn more or less coal as needed) but what happens when it’s a cloudy day or the wind isn’t blowing? Demand of course remains constant (we don’t turn off the fridge if the sun isn’t shining).
This makes it very hard for the utility companies because it requires significantly more forecasting capabilities, more flexibility to switch from one resource to another, and a more complicated and decentralized transmission system, etc.
Simply put, there are a lot more variables to control in a RE system and hence, more potential risk and volatility.
The industry term relevant here is capacity factor. This looks at the how much energy is actually produced by a specific source divided by the total capacity of that source. For example, if a 100MW solar plant produces an average 20MW (this is both due to efficiency of the solar panel and because the sun doesn’t always shine), then it has a capacity factor of 20%. Non-RE sources, because they are available on demand, obviously have a significantly higher capacity factor and the losses are only due to efficiency, maintenance, etc.
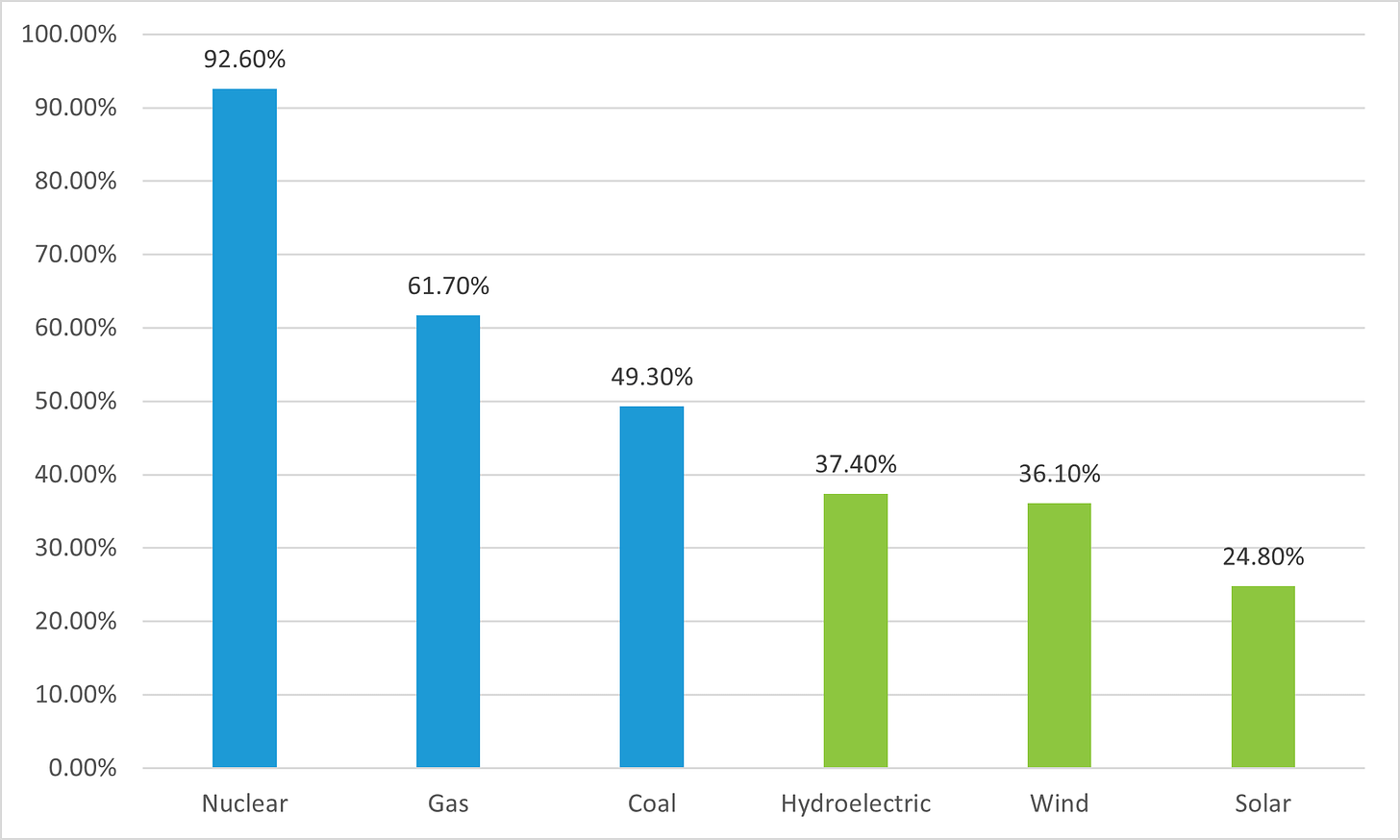
Technologically, there are multiple ways to fill that demand-supply gap.
Backup FFs: The most obvious way is to just have thermal generation power plants (coal or natural gas) ready on standby. They can step in whenever RE supply decreases. This happens in a lot of regions with high RE capacity.
You can see below how natural gas picks up when RE (solar in this case) drops off.
Source: Loth et. al (2022) Storage: The RE solution is to of course have more storage capability. Ideally, this would mean that we install excess RE capacity so that when the wind is blowing and sun is shining, supply exceeds demand, and the surplus gets stored. Then when demand exceeds RE supply, the storage plugs that gap. Storage can be in various forms, but the two most popular ones are likely batteries (of which there are also many kinds) and pumped hydro (kind of like a dam). Other technologies include hydrogen and bioenergy, but those are for another time.
Flexible demand: This is increasingly becoming popular as well. In an increasingly electrified world with smart appliances, we can potentially reduce demand when the supply-demand gap becomes too big. For example, ensure that not everyone charges their EV at the same time, or offer consumers discounts to run their washing machines when overall demand is low, etc. Industries can participate in this by offering to lower their consumption during crunch periods (e.g. when there is a snowstorm and its more critical for hospitals to get electricity than an aluminum smelter). By the way, this applies to FFs as well because storms, outages, etc. can happen to any system.
So when people do RE analysis to solve the reliability problem, there are multiple choices and nuances that can change the result:
How much storage is required? This is very location dependent. Different regions have different susceptibility to weather volatility — both in terms of length and frequency — and other factors such as changes due to climate change, prevalence of storms, etc. A study that analyzed 35 years of the German power system found that storage that equaled 10% of total generation capacity, covering about 12 weeks of backup, was required to get through the worst years. That is 40x was Germany had installed in 2018 — a sizeable but not insurmountable task.
While that is for electricity (which is only ~20% of total energy consumption), there are additional technologies that need to be scaled up for more industrial energy demand.
How diversified is the RE portfolio? If solar and wind plants are geographically concentrated, then they have a high correlation in terms of facing bad weather. However, if they are spread across multiple locations, then there are better odds of generation from one location being able to cover reduced generation from another location. This, of course, is more costly as it requires more transmission infrastructure (more on this in the electrification piece) and lower economies of scale.
There is also the question of how many other non-FF technologies can be employed in the system (e.g. geothermal, nuclear, hydro) to serve as backup or support. Not all countries have the same resources available.
How much overcapacity can the RE system have? One thing is for sure — solar and wind systems will need considerable overcapacity to make up for the lower capacity factor. Remember, actual production is simply installed capacity x capacity factor. So if you increase the installed capacity, you can have more energy production. This has the additional challenge of "curtailment”, which means switching off RE plants when supply exceeds demand. Again, more planning, more infrastructure, and higher costs.
Oversized system means more energy throughout the day but needs to be clipped (curtailed) at peak hours to maintain system balance.
All this ultimately boils down to cost, which is the main thing that everyone looks at. The job of the energy system is to reliably meet demand at minimal cost.
We have all seen those charts showing how drastically cheap RE has become, which leads people to say why aren’t we just building more RE. But as explained above, there are multiple other factors that go into the energy system beyond just installing more capacity.
The cost that we typically hear about in the mainstream is the Levelized Cost of Energy (LCOE) — this is the cost of producing one more unit of energy. This, however, is not the full cost because it misses the cost of backup, intermittency, overproduction, etc. — all the things needed to make that extra unit of capacity work reliably.
The full cost is called the system cost and is what we should be actually looking at. These non-generation costs increase as more renewables get added to the system.
Here’s the chart (or a variation of it) everyone always sees and talks about.
Now let’s look at different attempts to estimate the system cost.
System cost analysis from 2013 for Germany:
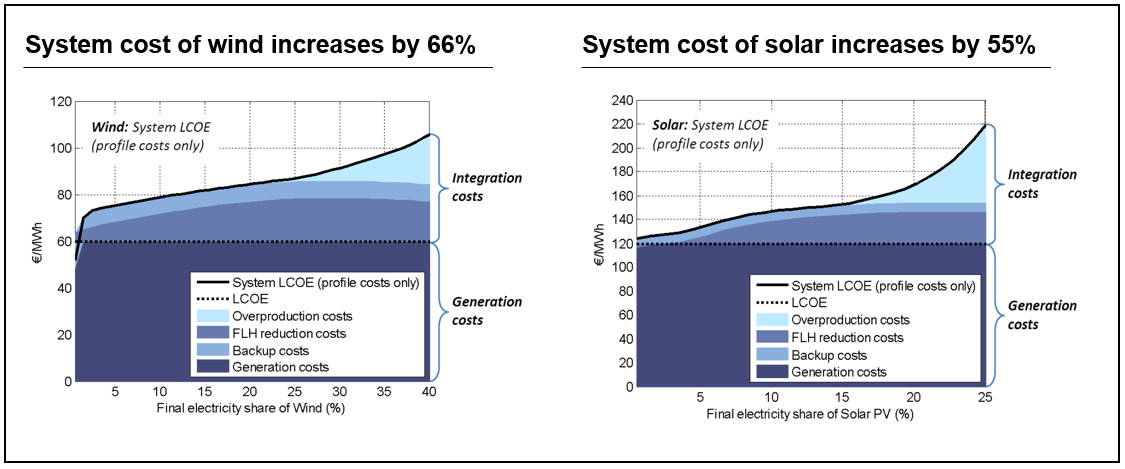
Cost of Valued Energy for Germany & California 2019-2021. This measure assigns a “value” to the energy produced by a source based on the price of electricity in order to account for the intermittency, curtailment, etc.
The green line represents the true systems cost. The cost benefit for RE seems to disappear!
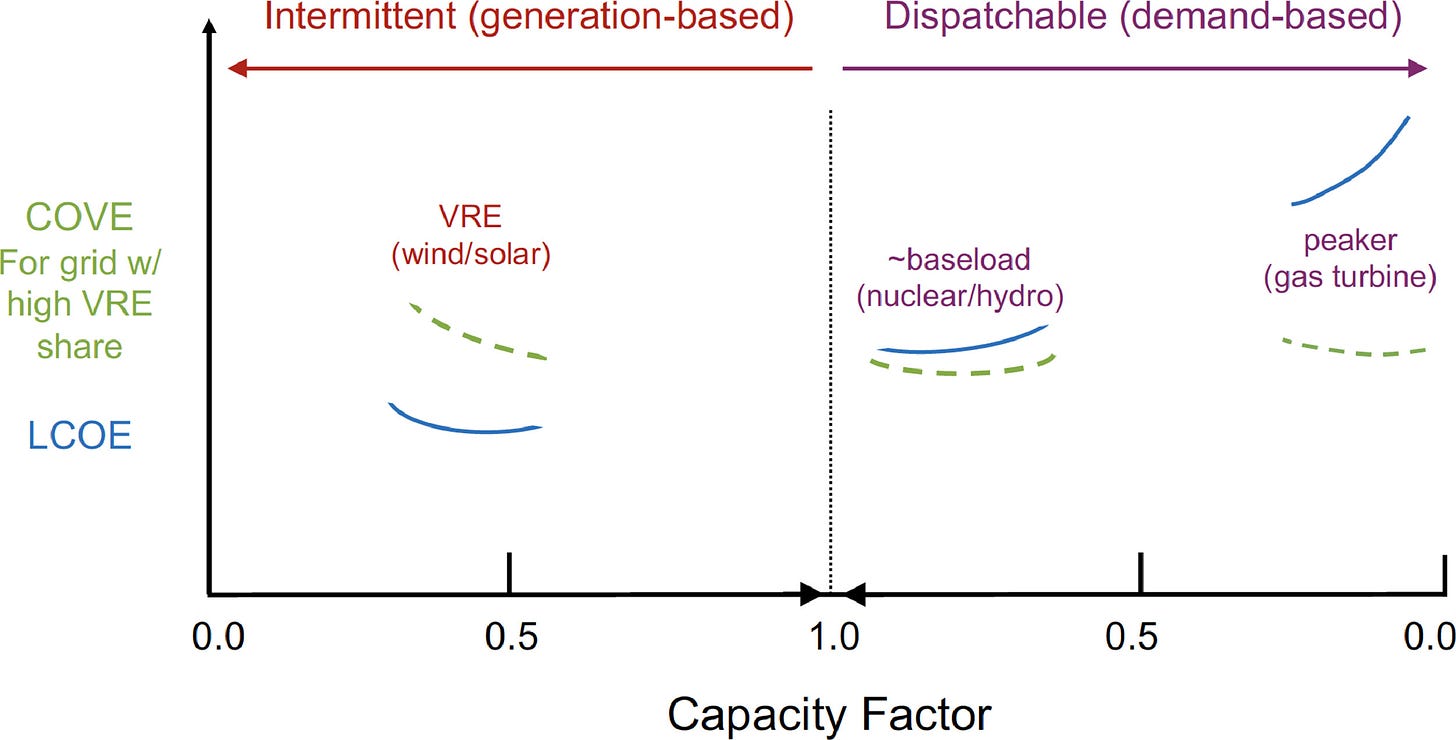
Levelized Full Systems Cost of Electricity for Texas and Germany 2012-2019. While LCOE assumes only generation cost, this metrics estimates the other end of the spectrum where it assumes that each energy source has to individually carry the weight of generation and system reliability.
You can see that the wind + solar model (W+S) is relatively comparable to nuclear but significantly higher than natural gas.
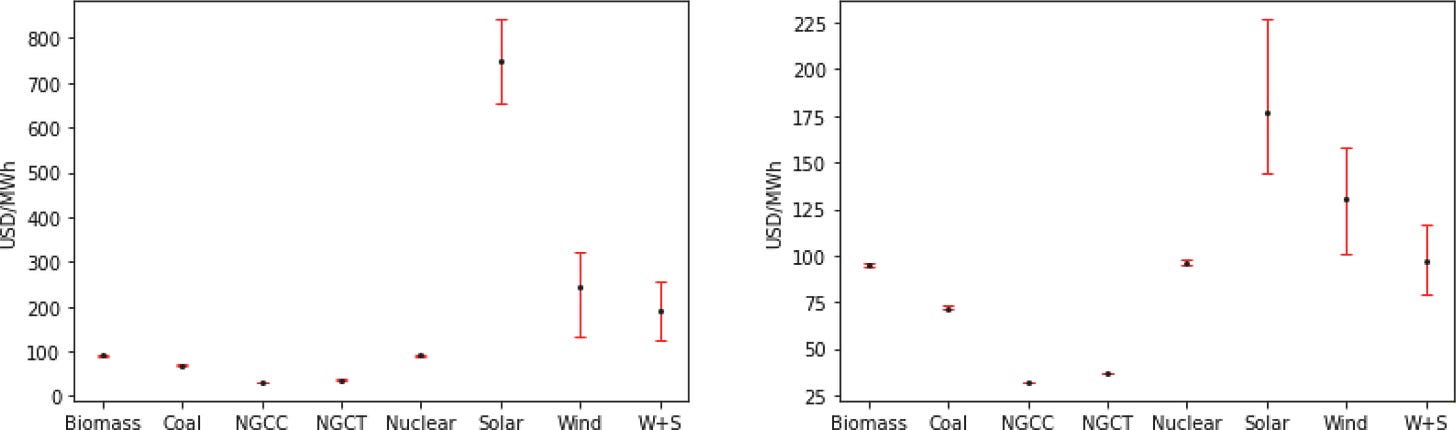
The takeaway here is both stark and critical. The mainstream narrative of cheap RE is grossly misleading because it only looks at the cost of generation, not of ensuring that the generation actually can be transported to its end-destination and used reliably. The real cost of RE is not as low as we think, which has serious implications for our civilization that relies on cheap and readily available energy.
2. Energy density
You may not have heard this but there is an easy mental model to understand density called the Iron Law of Power Density:
The lower the power density of an energy resource, the higher the material intensity.
There are multiple ways to compare density across resources, including surface area, volume, and mass. Across all 3 of those metrics, solar and wind rank considerably lower than fossil fuels. This is both intuitive and well-known as fossil fuels have had millions of years of high heat and pressure to compress large amounts of energy into small spaces.
[Note: Energy refers to capacity of work that can be done vs power is the rate at which work can be done. Energy is the size of the water tank while power is the much water can flow through the pipe.]
Power density by area of the generation site
Energy density by volume of the resource
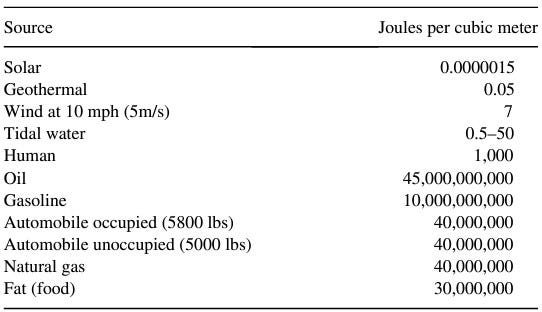
Energy density by mass (here RE are represented by Lithium-ion batteries)
However, when we think about land area, which is arguably the most important metric across the 3, the analysis above only looks at the power generation site (e.g. comparing a thermal power plant to a solar generation plant). That is misleading, and sadly what most anti-RE people use, because it doesn’t take into account the land area required for extracting the fuel (oil, coal, etc.), the area required for transportation (e.g. pipelines), and so on — called the lifecycle land area. When we take all that into account, the differences aren’t as stark.
A definitive answer on the land-use question is hard to come by. Estimates range from slightly lower land-use than the current FF system to 4-5x land-use. The important thing is to recognize the factors at play in different types of models:
How much does the new system rely on nuclear, geothermal, and hydro vs. just solar & wind?
What type of energy storage is being considered (mostly lithium-ion batteries vs. pumped hydro) because that impacts how much raw material will be mined?
Do we count the land area between solar panels and wind turbines as land-use for energy?
How much biomass energy (which is the most land-use intensive form because it’s basically growing crops like corn to be used for fuel like ethanol) is going to be used?
Lastly, the final 3 takeaways I would have on the land question are that:
1) It’s common to read analyses that say a very small amount of land could be used to power the Earth through wind & solar (0.3% of land to meet global electricity demand — which is only 20% of total energy btw) but these exclude the difficulty of transporting energy, ensuring reliability and so on. You can’t just put all your solar in one place, for reasons I explain below and in the next piece on electrification.
2) There are many unique solutions to reducing RE land footprint, ranging from agrivoltaics, covering canals with solar panels to reduce evaporation, using the land between wind turbines, etc.
3) Despite the lack of a definitive answer about the area needed for RE, the future land-use will look starkly different to the current system. Whether we can minimize ecological damage, social costs, etc. depends primarily on our ability to efficiently plan, collaborate and get things done fast enough.
3. Energy Return on Investment (EROI)
This piece is already quite long so I am not going to devote a lot of space to this because I think I covered what needs to be said in newsletter #2. In summary, EROI measures how much energy we get from a resource after subtracting the energy we spent to be able to utilize that resource.
For example, how much energy do we get from burning oil after we account for the energy spent extracting, processing, refining, and transporting it. That energy surplus is the basis of civilization. If we don’t have an energy surplus, then all our energy is simply spent getting the next unit of energy; there is nothing left for growth, development, and progress. This is what agrarian societies were for example: most of their time and energy was spent growing food for sustenance, so there wasn’t anything of those resources left for progress. Until of course we found ways to do more with less.
There is a lot of debate in the industry about what is the true EROI for fossil fuels vs RE. Again, a lot depends on how you set up the problem (e.g. what goes into the energy input required to use oil and how can we best measure that input).
From my own research into this, the majority expert view is that:
There is a minimum EROI threshold that needs to be met for our civilization work. This intuitively makes sense — most of our system is not spent in the process of extracting the next unit of energy, leaving considerable time & energy for us to do other things (all though it’s a shame most of us spend that doing non-creative jobs).
RE EROI < FF EROI. This means that we are moving from a world with high surplus energy to low surplus energy. More of our resources will have to be spent in getting the next unit of energy, making everything else harder and more expensive.
Here are two great resources that explain this better than me:
Does Renewable Energy Have a Higher EROI Than Fossil Fuels? - Art Berman
III. What’s in a name?
I mentioned at the start that the biggest driver for solar & wind is the decarbonization narrative. This is why these resources are called “renewable energy” or “clean energy” or “green energy”.
But are they really?
It shouldn’t be controversial to say that renewable refers to that things that can regrow themselves. Plants, for example, can be considered renewable because when they die, their remnants feed the cycle of growth for new plants with minimal intervention. Solar and wind, however, do not work this way. They follow the same cycle we have for everything else:
These energy sources are not renewable, they are rebuildable. A key distinction.
And as I said in the raw material piece, decarbonization = re-materialization.
Lots of RE analysis do another sleight of hand, similar as the cost narrative, where they don’t count the lifecycle ecological impact of solar and wind — the emissions from extracting materials, manufacturing, post-use waste, etc. Yes, the CO2 lifecycle emissions are much lower, but that just makes these resources “cleaner”, not clean (there’s a lot more ecological and health damage than CO2).
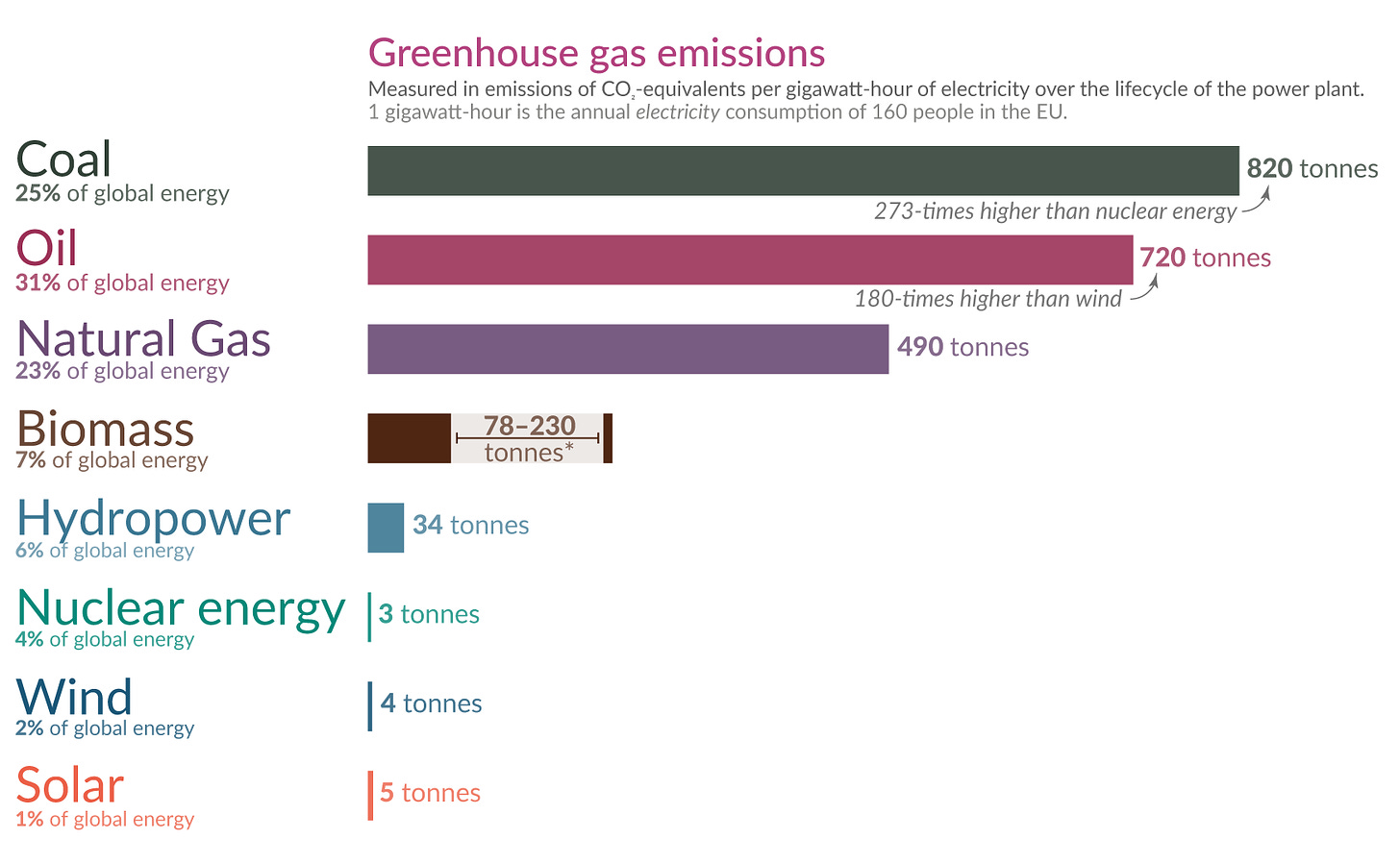
This is where the base layer foundation of this newsletter serves as a useful model. We know the cost and ecological challenges of the raw material value chain; we also know how the global manufacturing and transportation system is dependent on oil products (diesel, petrochemicals, etc.). So we can apply that to the simple fact that RE needs a lot more materials to produce energy!
Note: Add to this the materials required to (re)build our energy infrastructure to intergate RE, the machinery and equipment required for these sources to work (e.g. EVs, retrofitting buildings with electrified HVAC), etc.
“Take copper, for example: the world has mined about 700 million metric tons of copper since we started mining thousands of years ago. We’ll need to mine another 700 million metric tons just in the next three decades, Papathanasiou says, in order to meet climate targets.
Production of dysprosium and neodymium, rare-earth metals used in the magnets in wind turbines, will need to quadruple over the next several decades.” — MIT Technology Review
The materials exist, the question is how clean and green is it to extract them? Yes, the CO2 emissions of RE is considerably lower than fossil fuels. But what about the overall ecological impact of mining, refining, processing, and waste? What about the socioeconomic costs from indentured and child labor being used across the global mining sector?
Let’s say in the next 10 years we somehow create a 100% renewable energy system. The climate and ecological impact from using coal, diesel, gas, biodiversity loss, pollution, ocean acidification, etc. is not negligible. Some would say yeah that’s a cost worth bearing because the current system is worse, which is true.
But then in 2050, we have to do it again — rebuildable. How much of the energy and materials will come from the green and yellow lines in the above diagram? Can we recycle 100% or close of the materials? Those are all the questions we need to answer to determine how clean or green solar and wind will be.
The 3 takeaways here are:
RE is unequivocally better than FFs. However, the clean/green/renewable narrative hides the reality of the ecological damage that will still happen.
The level of green-ness of RE depends a lot on our choices. We could follow the existing model of ramping up coal to produce steel to produce wind turbines or ramping up cobalt production in Congo using child labor.
It’s not just about the scale of what we need to do but the speed at which we need to do it. To repeat, we need the same amount of copper in 30 years that we have mined across human history! (Yes, I recognize that replacements such aluminum, and recycling, offer an easier alternative, but this gives a directional sense of scale.)
Why this matters
Everyone is talking about renewable energy. But few understand the specifics and the nuances, which leads to 2 risks:
Losing the narrative war to pro-FF shills because RE people get caught out through their simplifications.
Getting hit in the face by ecological damage that we ignored or didn’t anticipate.
Renewable energy is an imperative, but it is no panacea. The sooner we realize that, the better it is for our civilization. One way or another, our global system is going to radically change, so systematically understanding how things work beyond the feel-good narratives is the only way to prepare for that.
There is a lot of positive news coming out about RE (like the one below), but hopefully this piece helps put all that in perspective. There is no energy transition yet, just energy addition!
Good analysis. Two things that come to mind, and as I’m a new subscriber, please forgive me if you’ve covered these sorts of things before.
Firstly, Degrowth needs to be considered, throw this GDP and growing economy shit out the window. Ok, ok, I know, it’s utopian and unlikely to happen by choice, but one can dream...
Secondly, I think it needs to be understood that the climate is driven not only by CO2 but also by LAND USE. Land use determines the water cycle, and if you clearcut a forest you destroy the evapotranspiration in that place and with it the cooling effect of the forest. The effect is fairly immediate. This was established by Millan Millan and was once well known but the IPCC, industry and governments latched onto the CO2-only narrative. It’s a bit pesky to consider that land use contributes to the climate when you want to put solar panels all over the place. This will affect the water cycle and climate.
A good place to read more about the LAND USE side of the climate is in this 3-part essay:
https://open.substack.com/pub/theclimateaccordingtolife/p/millan-millan-and-the-mystery-of?r=fhgru&utm_medium=ios&utm_campaign=post