#13: The electricity grid: A modern wonder of the world
Why the grid is at the heart of modern civilization, how it works (supply, transmission, and demand), and the major opportunities and threats as we race to reinvent it.
[Author’s note: This is the follow-up piece that was mentioned in newsletter #11 on renewable energy. While this explains the electricity grid, if you are interested in solar + wind and/or the price of electricity, I would encourage reading that piece first.
Over the next week, we will also be publishing a “year in review” piece for 2023, highlighting the main developments that happened, how our work potentially helped you understand them, and big things to look out for in 2024. So stay tuned for that, and, as always, please help share this publication with your networks!]
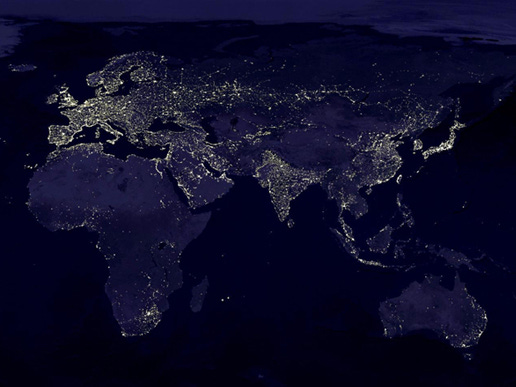
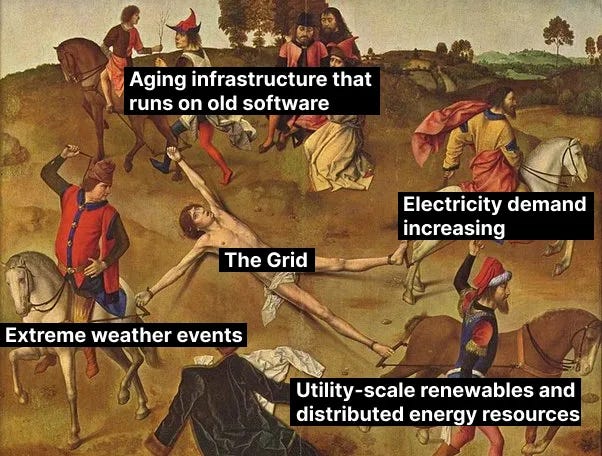
Image credits:
Perhaps one of the greatest and most underrated achievements of modern civilization is the electricity grid. Most of us don’t realize the scale, complexity, and coordination that is required to make sure that people have access to cheap and reliable electricity. Those of us from the Global South are much more cognizant of this because oftentimes electricity is either not always available or is expensive — or both. Over the past 2 years, richer nations have also begun to reckon with this as natural disasters (e.g. wildfires or extreme cold), higher energy costs, and mechanical failures due to aging infrastructure have disrupted the electricity grid.
We really cannot overstate how important the grid is as a topic going forward. It has all the 3 elements that make a topic exciting and critical:
Various, intersecting interests: Poverty alleviation, (re)industrialization & onshoring, climate change, national security, socioeconomic justice, etc. are all heavily dependent on an effective grid.
Multi-disciplinary: Modernizing and running the grid requires everything from engineers, manufacturers, innovators, financial traders, policymakers, and everyone in between.
Huge opportunity gap: Despite its importance, the grid has been a largely neglected sector for many decades, and hence is ripe for change and investment. There are a lot of quick wins and long-term disruption at play here.
Just to reiterate on the climate front, there is NO decarbonization without a radical transformation of the grid. (Newsletter #11 offers a deep dive on renewable energy, which should be read in conjunction with this piece).
While there are a lot of ways to look at the grid, this piece provides an introduction to electrification and explains the physical infrastructure that constitutes the grid.
I. Introduction to electrification
Electricity is one of the forms of energy that we consume today, accounting for 1/3 of global energy consumption. Of that, 66% is consumed by the two largest sources of electricity demand: industrial uses and residential customers.
Total electricity consumption has been growing at ~3% annually, which is close to the same rate as global GDP growth. That is to be expected as we have already seen in previous pieces how energy consumption is highly correlated to global growth. Most of this growth is being driven by Asia (largely India and China), but there are still huge gaps in the per capita consumption differences between rich nations and the Global South.
There are 3 main drivers of increased electricity demand going forward:
Fulfilling development needs of the world’s poorest: Despite progress and electricity being a requirement for basic necessities, there are still ~750 million people globally without access to it — ~600 million of them are in sub-Saharab Africa (50% of the population!) and ~100 million in Asia (places like India, Pakistan, etc.). Access to running water, light, basic heating/cooling, and other necessities are all severely impeded without electricity, which then impedes education, safety, economic activity, etc.
It is important to note that simply access isn’t everything.
There are ~1-2B+ people globally that have limited access to electricity or have to reduce consumption due to high costs. Take Pakistan for example. Officially, 95% of the population has access to electricity. But access simply means being connected to an electricity source, not that electricity is actually available. Even large urban centers have been facing up to 12 hours a day without electricity over the past few decades.
On top of that, the per unit cost of electricity in Pakistan is almost the same as that in the US (~$0.2/kWh) while the average salary in the US is about 20-30x times higher!
Decarbonization: Electricity demand is expected to be 75%-150% higher than today by 2050 (the bigger number is to achieve net-zero targets). That is a huge increase given we already have a considerable amount of electrification today. But given the scale of decarbonization needed, and that so many of the solutions (e.g. EVs, heat pumps, green steel, etc.) are electricity dependent, this shouldn’t come as a surprise.
AI, AR/VR, and other new tech: There simply isn’t enough recognition of how much electricity is consumed by technology. Even before this recent round of next-gen tech, things like video gaming and social media are already consuming high amounts of electricity. It is estimated that 2.5% of residential electricity consumption (~5-6% of total electricity consumption) is due to video gaming in the US — that is more than what many large Global South countries consume for their total electricity demand!).
Now with the increased proliferation of AI products like ChatGPT, we can expect exponentially increasing electricity consumption because of the increased data center requirements. Some estimates put just AI-driven electricity consumption at 3-4% of global electricity use by 2030! Now add to that AR/VR and other GPU-driven, data center-dependent technologies and you get the point.
So one way or another, our insatiable global system is going to need a lot more energy in the form of electricity going forward.
II. Understanding the grid
The electricity grid has evolved over the past 150 years owing to changes in technology, increased coverage in terms of area, and regulatory changes; however, the core principles have remained the same. All types of grids have three main steps: electricity generation, transmission & distribution, and consumption.
The goal of the grid is simple: reliably deliver electricity at the lowest possible cost.
How it does that is also simple: move electricity from where it is generated to where it is consumed.
One of the main reasons the grid is such an incredible achievement is that everything from large factories and buildings to small things like bulbs and phone chargers are connected through this massive network of wires and equipment. Given that this is not localized but covers huge cities and even whole countries, the task of keeping this whole system in balance is truly remarkable.
Simple visualization
More realistic and complex visualization (as generation and consumption nodes are spread out, not linear)
Just to recap from newsletter #11, supply must match demand at all times, by the second. Although demand follows a relatively predictable profile during the day, with predictable changes by season and other external circumstances, it still changes dynamically in real time. Everything about the grid is designed to make that work.
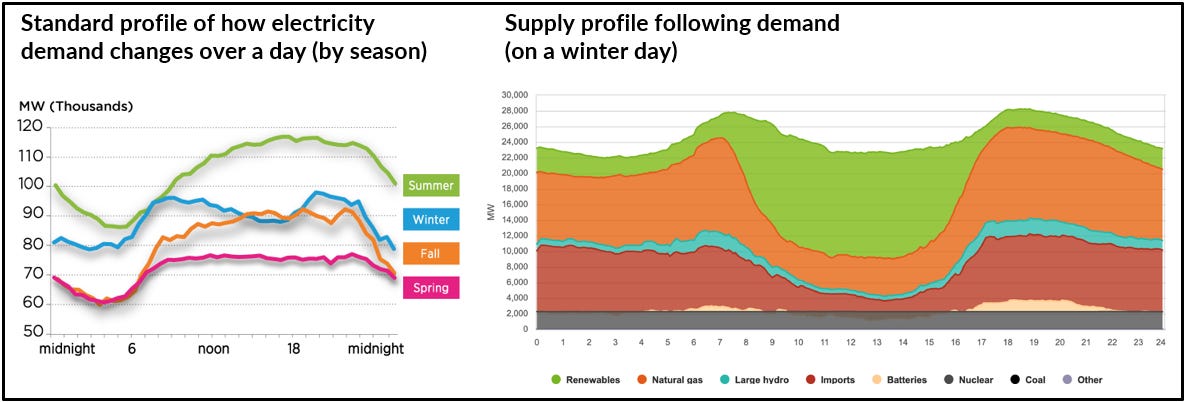
There are two main dimensions to the grid:
The physical infrastructure (generation, transmission & distribution, and consumption)
The operational infrastructure (the people and systems that make sure everything is in balance and working)
In this piece, we explain the physical dimension.
III. Electricity generation
There are various ways to produce electricity, ranging from huge power plants burning large amounts of fossil fuels or relying on water from dams to a small, single solar panel. You can see below that today, most of the electricity generated globally is through a combination of FFs and hydropower.
[The map below is a great visualization of electricity and socioeconomic development. Check out this amazing interactive tool to explore the power plant database.]
Not all generation sources are created equal though in terms of the value they provide to the grid. Remember, the goal is to make sure that supply equals demand at all times at the lowest cost possible. Demand is what changes first, and so supply has to follow — in real-time!
There are 3 types of generators:
Baseload: These are power plants that consistently provide electricity 24/7, with limited ability to ramp up or down. Typical examples of these are coal-fired plants, hydropower, nuclear, and geothermal.
Intermediate: These power plants are more flexible than baseload and are used to ramp supply up or down as demand changes throughout the day. Examples of this are combined-cycle gas turbines (increasingly the most popular), thermal powerplants using steam turbines, etc.
Peaker: These plants are used in rare instances (e.g., peak summer days) to avoid grid shutdowns and blackouts.
To understand the differences between different power sources and their qualities, let’s explore the 5 dimensions that matter:
Marginal cost: The cost to generate one additional unit of electricity, which depends on the fuel, operational, and maintenance costs. This is what is used to prioritize power plants on a day-to-day basis in terms of which gets used first to meet demand (cheaper ones go first). The graph above is stacked based on marginal cost. RE is missing from that graph but the marginal cost of production from wind & solar is zero as there is no fuel cost.
Capital cost: The capital investment required to set up the power plant. There is an inverse relationship between capital and marginal costs, which is why nuclear power, for example, produces the cheapest electricity when operational but has the highest capital investment required.
Start-up time and cost: The time and cost it takes to turn on the power plant after it has been shut down. Large thermal plants and nuclear plants can take up to 1-2 days to restart. This is why some plants are kept running even if they are not producing the cheapest energy (e.g., gas steam turbines run throughout the day even when surplus solar and wind is available).
Reliability / Capacity factor: This refers to the ability to generate electricity when needed (called “dispatchable resources”), as opposed to being intermittent (e.g., wind and solar). One key metric that captures this is the capacity factor: actual electricity produced over time as a % of the total capacity of the plant. Other reasons reduce the capacity factor as well, e.g., efficiency losses, maintenance downtime, etc. but intermittency is a major reason for solar & wind scoring low here. For example, solar panels have a 25% capacity factor, meaning that a 100MW solar plant produces 25MW electricity over a year.
Emissions: The intensity of CO2, SO2, and other greenhouse gases per unit of electricity generated over the lifetime of the source.
Power generation is currently evolving from a centralized industry to a more decentralized, distributed network. By having more generation nodes with an increasingly broad array of features, the grid is becoming substantially more complex. Most of the implications are on the transmission and operational infrastructure, but there are some key ones related to generation as well.
Let’s look at California as a case study.
It is a global leader in renewable energy, achieving the goal of running on 100% renewable energy some days of the year in 2023. Despite the remarkable investment, the conducive weather, and being home to Silicon Valley, natural gas still dominates the grid.
One of the biggest reasons for that is the infamous duck curve. The curve below (right image below) plots net-load, which is the total electricity demand minus renewable energy generation, for the best day of the year (when RE can cover the maximum % of demand). Therefore, the y-axis represents the power that needs to be generated by non-renewable sources on the best day for RE.
As solar is the most dominant renewable source in California, you can see that as the sun comes out, solar provides most of the electricity that is required. So for the time before dusk and after dawn, non-renewable sources run the show. The interesting thing is that even as renewable energy installations have been ramping up over the past 6-7 years, the belly of the curve is gone to 0 but the two ends remain robust. So even with more renewable capacity, the same amount of non-renewables still need to be installed and available from dusk to dawn, even on the best day.
On other, more typical days, you can see that natural gas is prevalent throughout the day, even when solar is at its peak. There are two main reasons for this:
We know natural gas will be needed from dusk to dawn. However, it is costly and impractical to rapidly ramp down these generators when solar takes over, and then rapidly ramp them up when solar dies down. Therefore, a certain amount of natural gas generators need to be kept running throughout the day.
Natural gas power plants need to make a certain amount of money to stay installed. Often, they cannot make that amount just by running from dusk to dawn; therefore, they are “selected to run” (will be explained in the operational infrastructure section) so that they make sufficient revenues to cover their costs — primarily capital costs.
California is an excellent example of how simply adding more renewable energy capacity doesn’t solve the problem, and has severely diminishing returns because if it is mostly solar, then it is tackling a specific set of hours daily — unless there is a lot more long-term storage that becomes available.
Lastly, and this does not get talked about at all, there are also severely diminishing returns when it comes to reduction in emissions, which should be the most straightforward benefit of RE. For all the reasons explained above, the reduction in emissions has plateaued despite the massive increase in installed RE capacity. After a certain level, RE just ends up displacing other low-emissions sources like hydropower and nuclear — which is the case in California — because those natural gas power plants are still required for their flexibility.
The bottom line for this section is that it is indisputable that we need more electricity coming from RE; however, even from a generation standpoint, the logic of how the grid works doesn’t always lead to the implications and benefits most people — including the most ardent RE supporters — expect. There is a variety of factors that come together to make electricity generation reliable and feasible, all of which have to be balanced within the realms of physics and engineering. To even get started with a real energy transition, these real challenges can’t be glossed over.
IV. Transmission & distribution (T&D)
This is the most interesting, overlooked, and arguably the most important part of the grid. The basic role of this part is to move electricity from the point of generation to the point of consumption. Transmission refers to moving electricity over long distances (e.g., tall electricity towers along highways), while distribution is the next step of moving electricity within specific localities to the point of consumption (e.g., electricity poles within neighborhoods) — the difference between a highway and a within-city street.
This is the level where the complexity really hits because it is an incredibly vast network of wires and cables of different sizes connecting hundreds of millions of consumption nodes (households, buildings, factories, etc.), generation nodes (all the power plants), and the T&D infrastructure (transformers, etc.)
You can see why T&D is going to attract significant investment going forward. There is no point in all the conversations around electricity sources and diversifying the portfolio if we can’t move that electricity to where it needs to be used. And with an increasingly distributed and decentralized grid, that is a tall order. How easy is that going to be in societies with increasing distrust of regulators, bureaucrats, and political office?
The key thing to reiterate before diving into this further is that if you think about the electricity coming into your home, it isn’t coming directly from the power plant. There is a web that intermediates this power flow and it is more accurate to think about the different zones of equipment that make it all happen. Therefore, each zone presents its risks and challenges. This is also why electricity is a fungible commodity — i.e., there is no way to tell where the electrons flowing into your house are coming from at any given moment.
Transmission lines
The first piece of equipment we will be focusing on is transmission cables. These are high-voltage cables that are carried by those big, pyramid-shaped structures we see along highways and open fields (although increasingly they are being placed underground). Their main task is to move electricity from the point of generation to the general point of consumption, which typically entails long distances, as efficiently as possible.
For the grid to work effectively, transmission cables need to mitigate 3 main problems:
Efficiency: Electricity flowing through cables encounters resistance, which results in power loss in the form of heat. This is exacerbated over long distances. Although losses are unavoidable, even small excess losses are a major loss of value when considering the scale of the grid. Therefore, transmission cables are designed to carry high-voltage electricity, as losses are inversely proportional to voltage (high voltage is like high water pressure, it pushes the electrons/water faster through the pipe). This is also why transmission cables are either placed atop high poles or underground, as the voltage levels of the electricity in them are deadly.
Currently, 4-5% of electricity is lost as heat during transmission.
Congestion: This refers to the fact that even though there may be sufficient electricity being produced to meet demand, the customer may not receive electricity because the transmission lines to that specific location are congested — another incredibly important point that most people don’t realize.
So for example, imagine demand is 1000MW and generation is ramped up to match 1000MW; however, the transmission line only has 800 MW. In this case, the customer will only receive 800MW and there will be power outages, even though enough generation capacity was available.
This is why the only way to think about the grid is as an intricate web of nodes all connected through T&D lines. Some demand nodes may have sufficient redundancy in terms of transmission lines, so even if one reaches peak capacity, demand can be served through other lines. For locations that don’t have this privilege, they either face power outages or high electricity costs (there are congestion charges).
This is also true from the generator perspective, as there are cases when the lowest cost generators are forced to produce below their capacity because the transmission lines they are connected to cannot carry more electricity, forcing higher cost (or higher emissions) generators to produce electricity.
Take the example below: at certain times, electricity prices in North & West Texas are negative (meaning the power producers would have to pay to produce electricity!) while prices in South & East Texas are not just positive but high. Both are losing out simply because there is not enough transmission capacity to carry the surplus wind energy from North & West Texas to the major demand centers in the South & East.
Safety: Either due to high air temperature or due to excess heat being generated when transmission lines carry too much electricity, the lines expand and sag, which makes them vulnerable to trees and other hazards. This can not just create a fault in the line and lead to power outages but can also spark wildfires.
Transformers
This is the second critical piece of equipment in the T&D infrastructure. We won’t go into too much detail here except to briefly explain what transformers do and the challenges we face in rolling them up with the required features, and at the required scale, that is needed.
Transformers are electromagnetic devices that are used to step up and step down voltage (this is for an alternating current system, which most of the world is). When generators produce electricity, it is at a low voltage. Before transmission, the electricity goes to a substation to be stepped up to very high voltages — for reasons explained above. At the other end of transmission, a substation uses a transformer to step down the voltage, which then makes the electricity viable for distribution.
Additional transformers are then used as part of the distribution network (the ones you see around your neighborhood) to further step down the voltage to household consumption levels.
It is important to stress how critical transformers are to the modern grid. Our increasingly electrified society includes use cases that require a broad variety of voltages — for example, household devices, commercial data centers, factories, etc. all use different voltages. Within each group as well there are voltage differences based on specific requirements. The one device that allows all these to be served is transformers. Therefore, without adding, managing, and upgrading transformers, there can be no reformation of the grid.
Lastly, as we integrate more renewable energy and the grid becomes more distributed, the role of transformers becomes more complex and important. A distributed grid simply means more transformers are required at various nodes, while a more diverse generation portfolio, coupled with rooftop solar, EVs, smart meters, etc. requires transformers with considerably more flexibility and voltage ranges. Without these features, ensuring that the grid is operating smoothly without outages and other risks (e.g., sparking wildfires, etc.) will be increasingly challenging.
This is precisely why over the last few years, with supply chain constraints, higher material costs, and the scramble to improve grid operations, transformer availability has gone from a few weeks to 1+ year, and prices have risen by 400%+. Without expanding manufacturing capacity for transformers, this is a serious impediment to the energy transition, domestic manufacturing, and national security.
To conclude this section, developing the T&D infrastructure of the grid is at a serious crossroads. On the one hand, the level of investment required is staggering, unlike anything human civilization has done before. The amount of T&D cables required globally to achieve net zero is the length from the Earth to the Sun, while the capital investment has to be 10x from 2022 to 2050!
On the other hand, the challenges in making this happen are vast. This is all physical infrastructure that needs to be built, but there are blockages at every step of the value chain:
Planning & permitting: It can take years to get transmission infrastructure approved because it requires coordination across land developers, regulators, communities, etc. For example, a 732-mile T&D project in the US West was approved in 2023 after 18 years — NIMBY-ism!
Manufacturing: Transformers, T&D cables, and other equipment are all resource-intensive, requiring massive amounts of copper, aluminum, steel, etc. All that has to be mined, processed, and then manufactured into the equipment, which typically happens across countries facilitated by seamless supply chains.
Implementation: Even with all the equipment ready, there are a lot of resources (labor, energy, etc.) required just to put all the poles, cables, and machinery in place. This is especially challenging for T&D because it is, by definition, spread out over vast distances, from densely populated urban areas to far-off, difficult terrains.
IV. Demand
This is relatively the simplest part of the grid operationally, but it is changes in this domain that are causing ripple effects through other parts of the value chain.
From the previous two sections, here’s what we already know about demand and how it is changing:
Demand is what drives the electricity grid. Generation must constantly match changes in demand, both in real-time (minute by minute) and over the long run (as the world becomes more electrified).
This is a broad category that encompasses an array of quite divergent agents, ranging from households and commercial buildings to heavy industry and data centers.
There are various drivers of increasing demand, ranging from reshoring manufacturing, providing electricity to those without it, electrification of society, etc. The demand from AI, data centers, and AR/VR is going to be a major factor. Most importantly, increasing extreme temperatures and climatic events are going to require considerably more electricity consumption (heating, cooling, etc.)
We are moving from static, predictable demand profiles to more dynamic demand profiles because of smart devices, EV charging, etc. Therefore, there are a lot more variables that need to be factored in to make more accurate predictions (which is what is needed to have power plants ready to go) and ensure that the grid is balanced (from the T&D side).
The most important feature that we have not covered yet is that, for the first time in history, demand centers are becoming bi-directional, meaning that they both consume and produce electricity. Rooftop solar and small-scale commercial solar plants used by industries are allowing consumers to have on-site electricity generation. This blows apart the fact that the fundamental goal of the grid has been to enable a single direction of energy flow from generators to consumers. Now, however, there are two complications:
Generators need to provide electricity that is net demand (total demand minus on-site generation). This creates even more volatility because generators have to both match changes in demand and changes in on-site generation.
The T&D infrastructure needs to accommodate electricity going into the demand centers and coming out of the demand centers. This creates multiple challenges because there are often voltage differences that need to be regulated, a potential overload of T&D infrastructure, etc.
To better manage all of the challenges above, demand centers will need to incorporate smart meters and other smart devices that can create and help create a digital grid. These devices allow for better transparency, more flexibility, and an increased ability to localize issues before they cause wider grid problems. This adds to the complexity for grid operators and adds to the infrastructure rollout challenges (same issues as T&D around equipment and installation).
Lastly, there is an interesting development in how demand centers are responding to how the grid is changing. In the T&D section, we highlighted that one of the big challenges was that large amounts of renewable electricity was left stranded and/or had to be curtailed (forced shutoff) because it couldn’t be moved to demand centers. Modular and mobile demand centers, such as data centers, Bitcoin mining, and small industrial applications like grinding, are moving their operations to where the stranded electricity is located.
This creates a win-win situation: the demand centers can get access to cheap electricity, while the electricity producers get to receive a positive revenue rather than be forcibly shut off. These demand centers are also innovating to provide more grid-stabilizing services, such as voltage regulation and shutting off when the grid is overloaded so the electricity can be repurposed for urban centers.
The latter point is called demand response, and grids like Texas have incorporated this recently by incentivizing Bitcoin miners to move to West Texas (with surplus wind energy). For most of the year, these miners consume the surplus electricity and create a win-win situation; for the few days of the year when demand is at its peak (the hottest days) or when supply is struggling (winter storms), these miners shut off and some of that surplus wind electricity can plug the gap.
To conclude, customers are moving from passive consumers to active participants in making sure that the grid is working well. Homeowners need to use smart appliances, rooftop solar, and EV charging in a way that is coherent with how the grid is evolving, while industrial customers are willing to relocate and have on-site generation. Apart from the infrastructure rollout, this requires new modes of coordination — as said above, that is a challenging ask in a fraying sociopolitical environment.
Why this matters
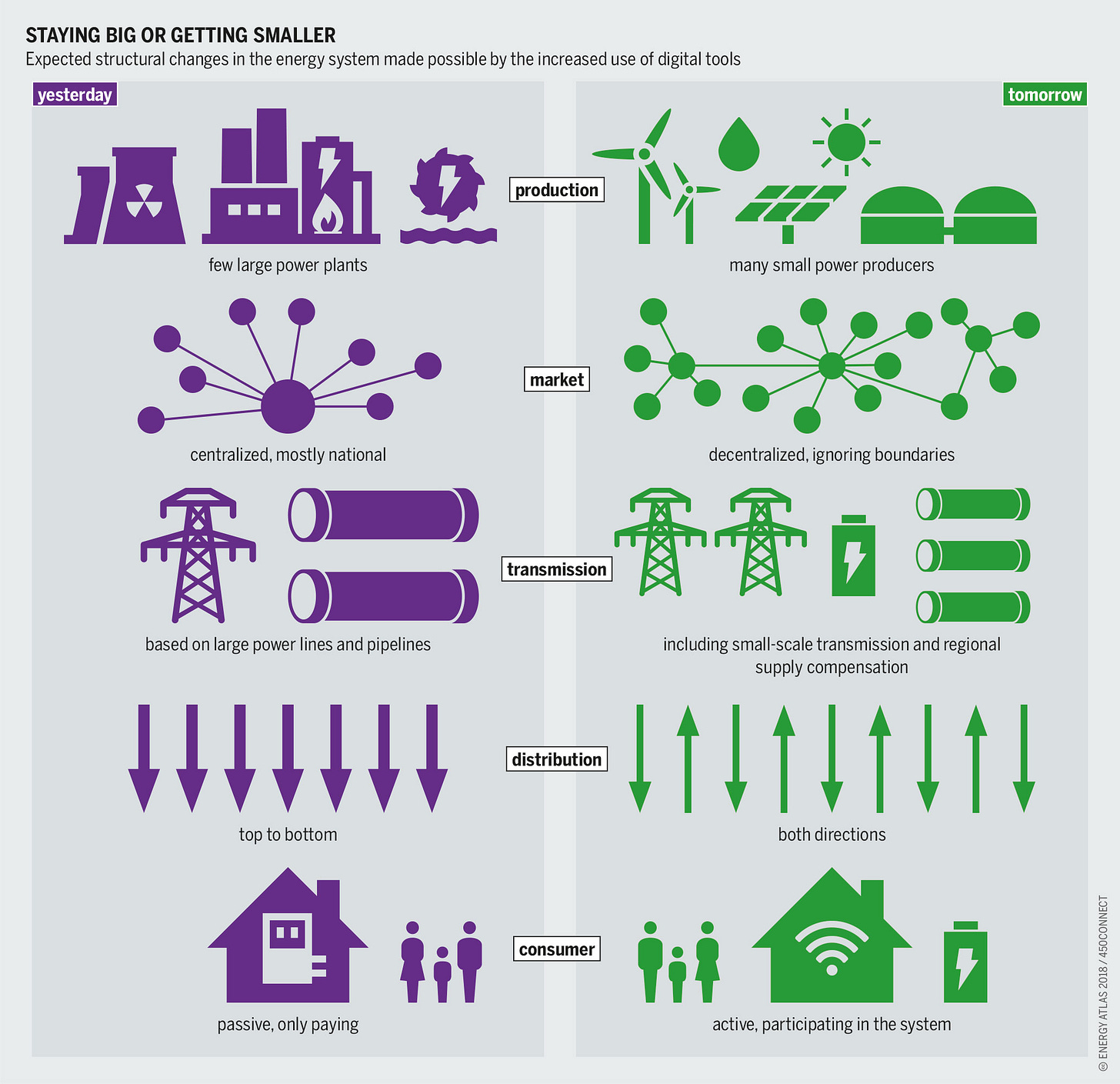
Hopefully, by this point, it is evident that the grid is at the heart of modern civilization. It requires the best of all human systems — physical infrastructure, real-time management, policy and regulation, and technological innovation — to work together seamlessly 24/7. While this piece explained the physical infrastructure, 3 important topics remain for anyone looking to think about and work on reinventing the grid.
Climate resilience: As ecological damage picks up pace, the grid is going to be forced to adapt and become resilient to imminent catastrophes. There are two ways in which the grid is affected by these events: increased stress resulting from higher and more volatile demand; and direct damage from wildfires, extreme heat/cold, flooding, storms, etc. Often, these occur at the same time and compound the risk.
Source: CNN Here are some examples
Winter storm in Texas: Freezing temperatures in February 2021 caused power outages across the grid as a lack of winterizing the natural gas and wind turbines were forced to shut down. Estimates of resulting deaths range from ~50-500, with a ~$80-130B economic loss. Electricity prices shot up from ~$25/MWh to ~$9,000/MWh, which customers were forced to pay. That’s more than what most families make in a month! At the same time, the extreme cold was forcing people to turn up their heat to survive.
Extreme heat in China, France, India, etc.: Record-breaking summer temperatures forced grids in these countries, amongst others, to ration power and adopt rolling blackouts. This resulted in tremendous human damage, particularly to the vulnerable populations who don’t have backup power or require medical services, and economic loss as industries are forced to shut down. This happened both because demand surged and high temperatures reduced electricity generation — solar panels have reduced efficiency at high temperatures, nuclear power plants shut down as the water they need for cooling becomes too hot (which happened in France), etc.
Floods in Pakistan: 1/3 of the country was inundated with water during the summer which led to power outages of 10-14 hours as ~25% of the electricity uses hydropower sources. There was also longer-term damage to the physical infrastructure, such as T&D poles, transformers, etc.
In short, measures such as replacing wooden poles with steel poles, undergrounding transmission cables, and waterproofing and winterizing critical equipment, are necessities now for a functioning grid.
Politics of the grid: There is a lot to be said here but there are two dimensions across which politics are much more important than most realize:
Domestic political setup: Grids vary from centralized, fully government-owned entities to a privatized value chain. We are not going to get into the pros and cons here, but the critical questions to think about are: how can electricity be provided to vulnerable and unprivileged populations? what profit-making motives should be in place given that electricity is a basic necessity? how does accountability work since all grids require some centralized coordination?
Ultimately, the grid suffers from the same politics that all critical services and commodities (e.g., healthcare and education) do. The additional angle is that given the physical nature of the grid, there are a lot of working-class jobs created by this sector, which raises questions about unionization and fairness. How do we make sure that the people who make the grid function have access to, and can afford, the commodity they help create?
Geopolitics: Ignoring the global supply chains for a second, there is a lot of electricity trading that happens across international boundaries. Imports and exports of electricity are important for grid balancing, avoiding blackouts, etc. Like with other forms of trade, they require trust and coordination. Unlike other forms of trade, the physical infrastructure required for these interactions creates the risk of huge sunk costs and leaves little room for real-time alternatives. As the grid gets more distributed, electricity trade will likely increase, which raises questions about co-owing the T&D infrastructure, balancing trade necessities with domestic necessities, etc.
Material reality: We have said this in other newsletters, and it is perfectly showcased in this piece — decarbonization is rematerialization. Even more so for the grid, the material requirements are going to surge higher even without the renewable energy angle because of all the reasons mentioned at the start. To learn about this in more detail, would encourage you to read newsletter #11 where we cover raw materials comprehensively.
In summary, the amounts of silver, copper, aluminum, steel, rare earth metals, lithium, and other materials that are going to be needed will require expanding mining capacity at a rate faster than at any other time in human history. We already consume a lot of these materials, and now we will have to consume substantially more, and at breakneck speed. That brings to the fore its challenges around the ecological impact of mining, the geopolitics of supply chains, the politics of manufacturing, etc.
Source: Deetman et. al (2021)
With that, we wrap this piece up. The initial plan was to also cover the operational infrastructure — how grid operators balance the grid, select generators, price electricity, etc. — but that warrants its own piece for the future.
Дякую, як завжди, Ви також забули додати, що газ, вугілля, нафту, уран - треба звідкись постачати, для цього треба залізниця, вантажівки та трубопроводи. Щодо відновлювальних джерел, - обмеженість місць генерації (вітер не дує в низинах, сонце марне вище 60Північної/Південної широти, тощо)